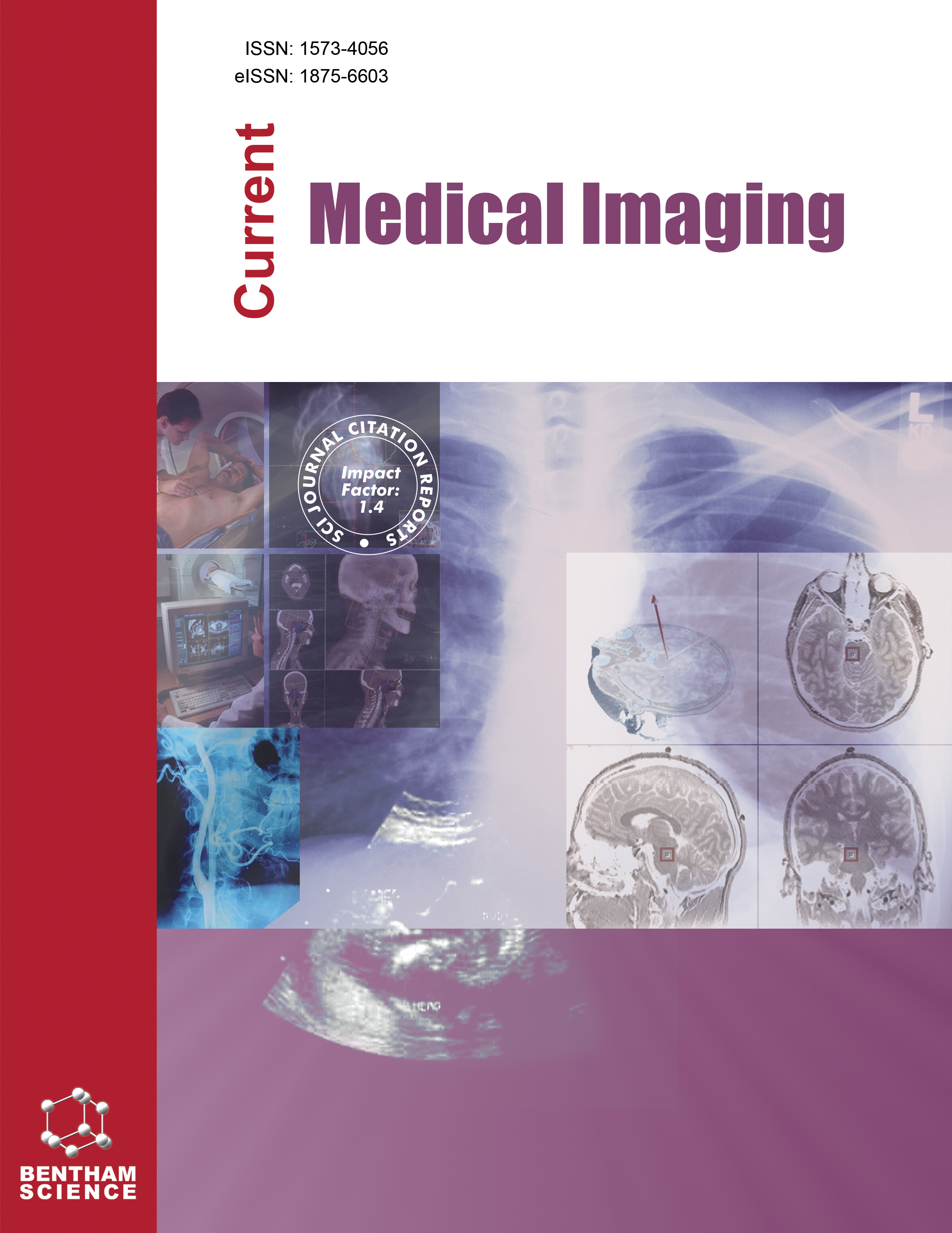
-
oa White Matter Fiber Bundle Alterations Correlate with Gait and Cognitive Impairments in Parkinson’s Disease based on HARDI Data
-
-
- 12 Jun 2024
- 29 Nov 2024
- 14 Jan 2025
Abstract
The neuroanatomical basis of white matter fiber tracts in gait impairments in individuals suffering from Parkinson’s Disease (PD) is unclear.
Twenty-four individuals living with PD and 29 Healthy Controls (HCs) were included. For each participant, two-shell High Angular Resolution Diffusion Imaging (HARDI) and high-resolution 3D structural images were acquired using the 3T MRI. Diffusion-weighted data preprocessing was performed using the orientation distribution function to trace the main fiber tracts in PD individuals. Clinical characteristics between the two groups were compared, and the correlation between the FA value and behavioral data was analyzed. Quantitative gait and clinical parameters were recorded in PD at ON and OFF states, respectively.
The mean tract-specific FA values of the right Cingulum Cingulate (rCC) were statistically different between the PD group and the HC group (p =0.047). The FA value of 34-58 equidistant nodes in rCC was positively correlated with Mini-Mental State Examination (MMSE) (r=0.527, p=0.024), Berg Balance Scale (BBS)-OFF (r=0.480, p =0.040), and BBS-ON (r=0.528, p =0.024) scores, while it was negatively correlated with the MDS-UPDRS-III-ON score (r=-0.502, p =0.030). Regarding the gait analysis, the FA value was significantly correlated with velocity, cadence, and stride time of the pace and rhythm domains in both ‘ON’ and ‘OFF’ states, respectively (p<0.05).
This study served as an initial exploration to establish that HARDI sequences could be employed as a robust tool for analyzing microstructural alterations in white matter fiber bundles among PD patients, although the sample size was small. We confirmed microstructural integrity impairment of rCC to be significantly associated with both gait and cognitive deficits in patients with PD. Early detection of microstructural changes in rCC and targeted treatment can help improve behavioral disorders. In the future, we intend to further integrate multimodal data with assessments of patient behavior both prior to and following intervention. We will validate our findings within an independent cohort to monitor disease progression and evaluate the efficacy of therapeutic interventions.